Basic Astronomy (Session One)
Solar System
Sun
Our Sun (called Sol by the Romans from which we get the word solar) is a main sequence G2 dwarf star. This means that it is fusing hydrogen into helium as its main power source, and that its mass is such that it emits its most intense light at a yellow frequency at about 5800 degrees Kelvin.
The Sun is a micro variable star. The Sun was once thought to be a constant star, but we have learned that every star varies to some degree. The Sun is in a state of "hydrostatic equilibrium" balancing between two forces, nuclear energy which heats the Sun and tends to cause it to expand and gravity which tends to compress the Sun. As the nuclear processes get the upper hand, the Sun expands. However this causes the temperature to drop which in turn slows the rate of fusion which is crucially sensitive to the fourth power of the temperature. As gravity in turn gets the upper hand, the temperature rises and the nuclear reactions begin to accellerate causing yet another cycle of expansion.
In a relatively stable star such as the Sun (a so called main sequence star), the oscillations are gentle. However this situation cannot remain stable for an indefinate period because the nuclear reactions use up hydrogen fuel which is not replaced. The "ashes" of this nuclear reaction - the element helium, is heavier than hydrogen and sinks towards the core. The reaction begins to leave the central core in a spherical shell the slowly grows closer to the surface. The Sun becomes somewhat denser throughout time and this in turn causes the energy production to accellerate. The Sun is currently about 30% brighter than it was when life first began on Earth. Life processes on Earth have maintained a roughly uniform temperature, but this has relatively little time left. The principle method of maintianing the temperature has been the sequestration of carbon dioxide, but our atmosphere has almost exhausted this process. In roughly a billion years the Sun will reach a point where it will be hot enough to cause the Earth's oceans to boil. This brightning has been occuring for just under five billion years and will continue for about another seven billion years.
As the Sun continues to evolve, the shell containing the nuclear reactions will grow large enough that the mass of the Sun above the shell will contain too little material to contain the "hydrostatic balance". At this point the Sun will swell violently, expanding past the orbits of Mercury and Venus and possibly incorporating the Earth in a large diffuse red star - a so called red giant. The Sun will continue to produce nuclear power from hydrogen until finally there is simply too little hydrogen left where upon the Sun will implode. Remaining hydrogen and helium will be heated to the point where an explosion will rip the outer shells of gas from the Sun leaving a very dense white dwarf remnant. We will discuss alternate paths that stars larger and smaller than the Sun will follow later.
The Sun has an absolute magnitude of 4.8 at the standard distance of 10 parsecs (2062648 AUs). This compares to an apparent magnitude -26.7 at 1 AU (8.3 light minutes). Of the hundred nearest stars only three Sirius, Alpha Centauri 1&2 are larger. Alpha Eridani is almost as large and then stars get small fast. Most are faint red dwarfs. The Sun has many features which have no counterpart in the planets. Here are some of these features:
The Sun emits a thin solar wind of particles driven from the Sun's surface by light pressure, electrical charges or magnetic fluxes. This solar wind can be detected throughout the solar system. Where these solar winds encounter magnetic fields in some of the large planets, these particles stream down along the lines of force causing auroras.
The Sun's corona is its very tenuous outermost atmosphere, an extremely hot gas which is in the millions of degrees. We normally do not see this gas, but during eclipses these pale outer atmosphere creates strange flame like images many times the size of the normal Sun's disk.
The chromosphere is the "atmosphere" of the Sun. It is much more dense than the corona (and much cooler) but it still is relatively thin when compared to the photosphere. The chromosphere absorbs certain frequencies of the Sun's light. Each absorbed frequency is specific to a particular type of atom. The pattern of alternating bright and dark bands (the Sun's spectra) gives us a great deal of information about the Sun's composition and chemistry.
The photosphere is what we think of as the Sun's "surface". When you look at the Sun through filters, it is the photosphere which displays texture. The photosphere emits the large majority of the Sun's light. This photosphere is not uniform. The Sun's surface has a mottled texture which reminds many people of the surface of a pot of cooking oatmeal. This granulation is the top of "bubbles" percolating up from lower levels in the Sun.
Sunspots are blotches which are slightly cooler than the surface as a whole. They would be brilliant if they weren't against the even more brilliant general surface. They increase and decrease in a pair of 11 years cycles (north and south hemispheres). During periods of intense sunspots, long range communications on the Earth may be disrupted. The area surrounding a sunspot is called an active region. Active regions are intense areas of magnetic flux.
Some solar storms create huge loops of gas along lines of magnetic force forming a prominence. These eruptions can be hundreds of times the diameter of the Earth. Solar flares occur when a granulation bubble breaks through the surface before it cools to the surface temperature. Material from the much hotter interior is exposed. Not only does visible light increase but so does the Sun's ultraviolet and x-ray radiation. Solar flares can be extremely disruptive. Sometimes the Sun's belches out a huge puff of electrically charged gas plasma. If this coronal mass (or discharge) happens to hit the Earth, power lines can be damaged, astronauts must seek shelter in the deepest parts of their spacecraft and aurora are intense.
The Sun generates its power in a central fusion core where the temperature is 15 million degrees Kelvin. Hydrogen gas is transmuted (changed) into helium with a great release of energy similar to the process in a hydrogen bomb. The Sun does not explode because its huge gravity hold the nuclear explosion in check. The radiation zone lies above the core. It is electrically conductive gas (properly called a plasma) transmits the electromagnetic radiation by direct radiation. As the radiation works its way outwards, it is progressively reduced in frequency from very short wavelength gamma radiation to x-rays and ultraviolet frequencies. The convective zone lies above the radiative zone and below the photosphere. This layer transmits energy by rotating vortices (bubbles). This "boiling" occurs in gas which is no longer so hot that it is a charged plasma.
Rocky Planets
The innermost planets in the solar system are formed with a central core surmounted by a rocky mantle and a thin crust (and a very thin ocean on Earth). Although we do not have a great deal of direct evidence, we believe that these worlds formed while the newly ignited Sun was propelling a titanic solar wind. Although the rocky materials and heavy metallic cores could form, a dense hydrogen and helium atmosphere similar to the outer gas giants was not possible.
All these worlds bear scars from the period roughly 4.5 billion years ago when they were formed out of the collisions of countless smaller bodies. Mercury presents a visual surface that is easy to confuse with the Moon. It is heavily crated. Earth bears definite crater marks, although the forces of weather and plate tectonics have erased many of these scars. We can see traces of craters on Mars and radar images of Venus reveal similar terrain. All this confirms that the early solar system abounded with small proto-planets that criss crossed the more circular orbits of what became the major planets.
Some of these criss-crossing worlds hit the planets and merged with them. We recently say Showmaker-Levy 9 revisit this process on Jupiter and we know that 65 million years ago, the dinosaurs died when a relatively small remaining proto-planetesimal struck the Yucatan Peninsula. Eventually only relatively large bodies in relatively circular orbits survived. Today, only Pluto is in a criss crossing orbit and it survives simply because it is in a strange 2 to 3 synchronous orbit with Neptune which always keeps them at least 1/3 of Neptune's orbit apart.
Mercury
Mercury, the innermost of the planets, shows the effects of it close proximity to the Sun in many ways. While it looks like the Moon as far as its topology goes, it differs from the Moon drastically. Its iron nickel core is a much larger percentage of its total volume. Mercury would also be the densest planet if it was as large as the Earth. Its gravity is too little to significantly compress its core.
Notice that the surface gravity of Mercury and Mars are both about 38% of the tug we feel hear on Earth. However Mercury's mass is only about half that of Mars. Why then is the surface gravity about the same? It lies in the fact that Mercury has a diamter only 72% that of Mars. If each had the same size globe, Mercury's gravity would be roughly half that of Mars. Gravity increases as the inverse square of the diameter. The inverse of 72% squared is just about two, with the result that half of two yields the same intensity of gravity. A similar line of reasoning explains why the Moon's gravity is about 1/6th that of Earth while its mass is only slightly more than 1/80th that of Earth.
It was once though that Mercury was tidally locked to the Sun, much the same way as the Moon is tidally locked to the Earth or the Galilean Moons are tidally locked to Jupiter. Tidally locked bodies rotate on their axis such that their "day" and their "year" are identical. Mercury revolves in synchrony with it orbit about the Sun in a ration of three Mercurian days in a Mercurian year.
The combined effects of Mercury's high eccentricity orbit and the planet's proximity to the Sun make it impossible to successfully use Newtonian physics to predict its position over long periods of time. The orientation of the orbit revolves slowly in accordance with Einstein's General Theory of Relativity.
Venus
The planet Venus has long been imagined as a paradise or at least an Eden. It great brilliance and its lunar like phases (suspected although not viewed since antiquity) made it a natural connection to the Moon. It is not coincidence alone that both bodies where associated with goddesses - Venus [Aphrodite] and Diana [Selene]. From the middle of the 19th century until about 2/3rds of the way through the 20th century, Venus was believed to be a damp, watery world, somewhat warmer than Earth but still a very likely abode for life. Numerous stories were written about this cloud covered world with people slogging through swamps or being besieged by rains as amphibian wildlife provided the local monsters.
The first signs that Venus might not be quite such a rainy swampland were the microwave signals from large radio telescopes. If the curves were to be believed, the temperatures weren't merely very warm but positively blast furnace like in intensity. This was confirmed when the first probes parachuted into a hellish world where the surface was hot enough to melt lead or tin, the air was as dense as water thirty feet deep , the clouds were boiling sulfuric (battery) acid, and water was no where to be found.
Venus had other surprises. It rotates backwards. Its north pole points in the same direction as the other planet's south pole. It has a extremely long day (18 of our days longer than its year). Weirdly, three Venerean days is just about exactly two Earth years.
The air is so dense that light is refracted completely around the planet. The cloud layer is so dense that daytime is only somewhat brighter than nighttime. If we could see much of anything on the surface, everything in the distance would seem red or orange because other frequencies of light are absorbed far above the surface.
Venus is just close enough to the Sun, so that a runaway greenhouse effect took place. Electromagnetic radiation is trapped beneath the cloud deck. When volcanos and crevasses open, any sulfurous gasses remain gas rather than cooling to a solid as they would on Earth.
Venus' apparent diameter changes radically from its farthest position to nearest point. At its most distant, it is about 1.8 AUs from the Earth. At its nearest it is about 0.2 AUs from the Earth. If we could see Venus at its very nearest, it would be 9 times as large as when it is at its most distant. Unfortunately, both these extremes occur when it aligns with the Sun.
You might think that Venus would be brightest when it was closest, but this is incorrect. As Venus approaches, it becomes an ever thinner crescent. As Venus approaches "full Venus" (ala full Moon), it also reaches its smallest disk. Venus is most brilliant at the point where it displays the greatest illuminated surface, a balancing act between its phases and its proximity to Earth.
Earth
When we are asked what planets are visible, we almost always forget to mention the single planet which is always visible, day or night. It is the one underfoot, the Earth. The planet Earth (called Terra by the Romans and Gaia by the Greeks) really should probably be called planet Water. About 7/10ths of it surface is washed by oceans, seas, lakes and rivers. Earth is a very high contrast planet, with brilliant white clouds against large blue areas and smaller orange brown areas. It has two very prominent polar ice caps.
Earth is not only the only known abode of life in the solar system, but a planet which has been radically altered by life. Our very atmosphere was manufactured by a mutant strain of bacteria. These strange bluish green bacteria started to break down compounds and emit a ferociously caustic gas oxygen. Most of the life on Earth died when in came in contact with this deadly poison. Today only in a few places where oxygen cannot reach do we find the survivors of this first and most dramatic case of air pollution. The mutant bacteria live on everywhere as blue/green algae. The scant survivors are the anaerobic bacteria found in hot springs and badly sterilized cans of food - botulism. You might think that life was basically a surface feature but you would be wrong. Our deepest wells and mines encounter bacteria many miles inside the Earth, living on whatever chemicals can sustain life.
Moon
Isn't it a bit out of place to call the Moon a rocky planet? Isn't the definition of a planet a large world which revolves around a star - our Sun? Certainly the classical definition of a planet was a large world rotating around a star like the Sun, but all sorts of intermediate and odd worlds are being discovered. Brown dwarfs often orbit stars but they are not really planets although they are very large. Asteroids can be quite large like Ceres [which was once called a planet] but they really aren't planets. Some large rocky worlds or gas giant world exist between stars if current ideas are correct. What do we call these? Aren't things which revolve around planets called satellites? Again the classical answer is yes, but once again we are finding all sorts of odd special cases. Some asteroids orbit each other. If the larger asteroid was called a planet, then wouldn't the smaller asteroid be its moon? Many of the outer moons began as asteroids. They have been captured when they passed the outer reaches of gas giants atmospheres or came too close to existing large moons of these planets. What are these bodies, satellites or asteroids? Pluto and Charon are so similar in size that they almost deserve to be called co-satellites The answer to all of these questions simply points out how very odd the Moon really is.
- The Moon is a large world, bigger than Pluto and not much smaller than Mercury.
- While the Moon circles the Earth, it always moves forward relative to the Sun. In fact the Sun's gravity controls the Moon's orbital shape about 3 times as strongly as the Earth's gravity. This does not happen with any other natural satellite. It is not adequate to compute the position of the Moon simply by treating the problem as finding where the Earth is and then computing an elliptical sub orbit for the Moon. All other natural satellites can be calculated this way for almost all purposes.
- Add to these two conditions the effect of tidal drag, and you get a world which is more difficult to compute than any other object in the solar system except for comets and asteroids which happen to come very close to large worlds.
The Moon is composed of a rocky mantle which is extremely similar to its co-planet Earth, but it lacks a metal core. [We can tell this through various tests such as a lack of a magnetic field and "moon quakes" which allow seismometers placed on the surface to allow to examine the interior as we do on Earth. Only one theory has survived rigorous computational modeling. The Moon must have been formed when the Earth was struck a glancing blow by an early proto-planet in an elliptical orbit. This proto-planet must have been the size of Mars approximately. Some of the material from the proto-planet and a great deal of the material from the Earth's mantle collected in a dense ring quite close to the surface. Eventually this coalesced into the Moon.
Tides
Throughout most of the world, there are two high tides and two low tides every lunar day (just under 25 hours). If gravity alone were the cause of the tides, there would be just one on the side of the Earth facing the Moon. In any case, the Sun would raise a much greater tide because the gravity of the Sun on the Earth is 832 times the force of the Moon on the Earth.
Tides are caused by an imbalance between orbital speeds at the center of a body and orbital speeds at the surface. Consider our diagram. Assume the body is following the gray line. The yellow dot at the center of gravity follows this orbit with neither an excess no a deficit of speed. However, the red outer particle is actually in a slightly larger orbit but still traveling at the same speed as the yellow dot. This means that the red particle is traveling slightly too fast for the orbit it is in. Left to itself the red particle would change its orbit's shape slightly creating a larger elliptical orbit. The situation for the blue dot is very similar, except that it is going just a bit too slow and would like to drop into a smaller orbit.
If the body were perfectly rigid, all that would happen is a tension at right angles to the orbit. However if the body is either completely fluid or covered with a fluid, a bulge is created on both the inward and outward sides of the body - a tide. On Earth, additional complications occur. First the Earth is spinning once in 24 hours (rather than just under 25 hours for the Moon). This means Earth is trying to accelerate the tidal bulge. This in turn exerts a breaking action on the Earth (the Earth's rotation is slowing a small amount every day and has been for billions of years). The loss of angular momentum must be matched by the laws of physics and the result is the Moon slowly recedes from us. A second complication is that the Sun also raises a smaller tide which is in a 24 hour cycle. This means that throughout the lunar month the tides sometimes reinforce each other and sometimes counter each other. This causes flood and neap tides.
The tides are not only raised on the Earth, but on the Moon. The Moon's tidal bulge is cast in concrete or more precisely in granite. The face we see is raised. If the Moon drifts to one side or another (a motion called libration), the Earth exerts a torque on the bulge and returns the Moon to face us.
The closer you get to a massive body the more severe the tidal forces on an extended body. Inside a certain distance (Roche's Limit), solid bodies cannot form. Would be satellites which venture too close to large planets become rings.
Extremely massive bodies with extremely small diameters (white dwarfs, neutron stars, pulsars and black holes) can have tides so strong that nothing can withstand them. An astronaut venturing too close to one of these monsters would feel his feet pulled into the black hole (or whatever) while his head was being wrenched off into outer space.
Mars
No planet has held a greater fascination for us than Mars. It is a place we might be able to live on if we provided ourselves with breathable air and some warmth. For those of us born before the space age, Mars was almost magical. It was the only planet with a surface that could be seen. At closest oppositions, it is quite possible to pick out polar caps, mountainous areas and plains.
However, Mars' image plays tricks on our eyes. The edges of two types of terrain seemed to be marked by long "canali". When Schaparelli used this term, it only meant "channel" in Italian but it wasn't long before the word was being called "canals". In turn, canals implied canal builders, and this in turn became ever more fanciful stories of dying races and desperate attempts to eke out meager water reserves from the polar caps for farms along the canals. Mars had other tricks to play on our eyes. Sometimes canals changed. We now know that these changes if there were seen at all were simply the result of dust storms covering and revealing the land below.
Mars is currently the target of a quixotic mission to send men to the planet. Whatever the social forces that prompt this, relatively little scientific information will be gained that could not be gained remotely.
Gas Giants
During the formation of the solar system, many planetesimals formed as whirls in a disk that spun around the proto-sun. Heavy elements sank towards the center of these bodies while the outer portions were wrapped in gasses - primarily hydrogen and helium. Once the Sun reached a point where its internal temperatures allowed it to ignite nuclear fusion, everything changed. The innermost planetesimals were lashed by extremely powerful solar winds which stripped away most of the hydrogen and helium. All the while all of the large planetesimals were accumulating smaller planetesimals eventually forming the eight large planets.
By now the innermost worlds were scoured clear of most of their atmospheres. Only denser and heavier gasses remained. On Earth, much of the hydrogen combined with oxygen to form water.
However, farther out the solar wind abated and the growing planetesimals could gather huge reserves of hydrogen and helium. So much hydrogen was gathered that it began to compress into unusual forms such as metallic hydrogen.
Jupiter
Jupiter is a planet of superlatives. Only Earth rivals it for markings. It outweighs all the other planets put together by at least a factor of 2. It spins on its axis faster than any planet. A jovian day takes less than 10 hours. Its "surface" gravity is more than twice that of the Earth. It has vast storms, larger in diameter than the Earth that swirl madly for hundreds (perhaps thousands?) of years. One such storm called the Great Red Spot has been continuously viewed since Galileo's time. Moving pictures from space craft dramatically show the clouds racing around the center at speeds of several hundred miles per hour.
Jupiter has a huge core of metallic hydrogen. Metallic hydrogen is hydrogen gas compressed so densely that it begins to behave like metals on Earth. In particular, it carries electrical current. This in turn creates a magnetic field which acts as a vast buffer between Jupiter and the Sun's solar wind. If we could see magnetic lines of force the magnetosphere would be larger in our sky than either the Moon or the Sun. Jupiter has huge lightning storms with bolts so powerful that its innermost satellites, Amalthea and Io are sometimes hit by them.
Jupiter volume is just about as large as it is possible for a body to be without becoming a star. If you dumped more material into Jupiter, its diameter would begin to actually shrink as gravity increased the density faster than material could be added.
It is a mistake to think that Jupiter is nearly big enough to make a star. It is nowhere close to that mass. It would have to be between 15 times its current mass to be a brown dwarf and 80 times its current mass to be the smallest main sequence red dwarf. In spite of not being able to sustain nuclear reactions in its core, Jupiter generates more than twice as much light (in the infrared spectrum) as falls on it from the Sun.
Saturn
When you think of Saturn, the words "the ringed planet" almost certainly jump to mind. While all the other gas giants have rings, none were discovered before the advent of space craft and some of them can only be detected when they blot out a background star as the planets passes in front. With Saturn there is no such problem. Saturn's Rings can be seen with the most modest tools.
The rings are swarms of tiny rock sized pieces of icy material. Long before the 20th century, astronomers and physicists knew that the rings where formed of countless particles. No rigid ring could have survived the tidal stresses on it.
Uranus
Uranus has to be the most featureless planet in the solar system. It is called green, but it is not the beautiful green of an emerald or an aquamarine but a pot of day old pea soup. You do not see the banding or cloud structures you see on Jupiter, Saturn or Neptune.
Uranus does have four substantial Moons, though none of them as large as Pluto. Perhaps the most interesting thing about Uranus is that its axis is tilted an extraordinary 98 degrees. It keeps its north pole pointing towards the Sun. Effectively, it has a warm pole and a cold pole, but differences in temperature are minimized by surface air flow.
Uranus was the first planet found by telescopes. This is a little odd because this planet is visible to the unaided eye under favorable conditions. However, it is so dim and unremarkable, that no one ever noticed it was moving slowly.
Neptune
Neptune is a deep blue with white wispy clouds and a dark blue spot similar to the Great Red Spot on Jupiter. Its orbit is nearly circular, with only Venus slightly more so. Neptune is the first planet which cannot be seen with the unaided eye. However, even the most modest binoculars can make it out as a faint bluish star, if you know where to look. At Neptune's distance, sunlight is almost a thousand times dimmer than on Earth. The Sun appears as a small disk, but could be easily mistaken for a extremely bright star.
Once we past Neptune, the solar system changes radically. The hydrogen gas required to form a gas giant thinned too much to form a ninth large planet. Only icy clumps of water, methane and ammonia which chunks of rocky planetesimals and dust were left to form bodies. Just outside Neptune's orbit various small icy worldlets formed. Much farther out where the Sun's gravity feebly contends with passing stars, the final layer of the solar system the cometary Oort cloud lies.
Ice Worlds
Pluto and Charon
AUs 39.529 Period 248.6 Orbital Vel. 4.7 Eccentricity 24.8% Inclination 17.15 Diameter 2200 Mass 0.17 Density 2.0 Rotation -6.387 Obliquity 118.0 Gravity 9% Escape Vel. 1
Beyond the orbit of Neptune lies a variety of icy worlds and planetesimals collectively called transneptunian objects. If these objects happen to approach the sun we would call them by a more familiar name - a comet. The structure of both Pluto and its satellite Charon are very similar in nature to the icy satellite of Neptune (Triton) and the transneptunian objects. In the late 1990s, the IAU made a move to reclassify Pluto as a transneptunian object rather than a planet. There were many reasons. Pluto is very much smaller than the other planets. It had a highly elliptical cometary orbit, actually coming inside of Neptune's orbit for a while. The plane of its orbit was well outside the plane of the ecliptic. It lacked either the rocky structure of the inner planets or the gaseous structure of the outer planets. The attempt to reclassify it failed when the wife of its Pluto's discover (Clyde Tombaugh) made an appeal.
When Pluto was first discovered, it was assumed to be a very large world. This was necessary if it was the cause of "perturbations" of Neptune's orbit that had been measured. In fact the "perturbations" turned out to be measurement and round off errors. A second reason that Pluto was thought to be large was its high relative brightness. This turned out to be a byproduct of its snowy icy surface (rather than duller rock or gas).
Satellites
Satellites in the solar system range from rocky planetesimals only a handful of miles across to great worlds larger than Pluto or Mercury. Some of these satellites had atmospheres and another seems to have a watery ocean. One satellite is white on one side and coal dark on the other. One has a huge crater that makes the satellite look like an eyeball.
Some small rocky and icy planetesimals of irregular dimensions end up as captured moons of the outer planets. Capturing an asteroid requires something to slow asteroid down such as a brush with the planet's atmosphere or a close pass by a large moon. Unless the excess speed of an asteroid can be discarded by brushing the atmosphere or by slingshot orbit changes around a large Moon, any incoming asteroid will simply shoot by a large planet.
When our first robot spacecraft reach the Jovian System we could not believe what we were seeing. We expected that the four Galilean Moons would be more or less alike. Astronomers had predicted they would be a rocky center with an icy covering. We will look at some of the large satellites, but the smaller satellites are worthy of study as well.
Io is a festival world of brilliant reds, and yellows, black smudges and white streaks. You say you don't like the way it looks, well wait a few weeks and Io will change - dramatically. It is by far and away the most tectonically active world in the solar system. Io turns itself inside out ever million years or so. It has active volcano's which spew sulfurous compounds onto the surface at a pace which far exceeds anything on Earth. It is as if the whole surface was like Volcano National Park on Hawaii.
If the surface wasn't wild enough, Io goes around Jupiter in a doughnut shaped cloud tube of ionized material (probably sulfur). It is as if Io has a strange atmosphere going all away around Jupiter.
Io has a really rough time being so close to Jupiter. Jupiter raises tremendous tidal forces in Io bending the rock and minerals back and forth. All this causes a great deal of heat from friction as materials rubs each other. Jupiter periodically blasts the surface of Io with great lightning bolts. The static from these flashes create the loudest radio noise in the 10 meter wavelengths.
Europa is the next major satellite out from Jupiter. It takes Europa twice as long to circle Jupiter as Io and half as long as Ganymede. Look at the surface of Europa. Those long lines are actually cracks, not in rock but ice. Mounting evidence suggests that this ice floats on a world wide watery ocean that in turn lies on a rocky core. If this sounds like our polar seas, it is hardly surprising.
While astronomers are fairly certain that Europa has some sort of an ocean, there are questions whether it is water, slush or some more exotic mixture with water and other materials acting as an antifreeze.
Ganymede is a very respectable world in its own right. While not as dense as Mercury it has a diameter which is greater. It has a surface which is three quarters of the Earth's land area. Ganymede is more like the Moon than any of the other Galilean Moons. It shows the type of cratering we see on both the Moon and Mercury.
Callisto is more like Pluto than any of the other Moons. Far enough from Jupiter so that tidal forces do not create frictional heat in huge amounts, it is the coldest of the Galilean Moons. Much of Callisto is icy material.
Titan, seconding size to Ganymede by a scant few miles, is another very substantial world. This moon is an orange brown color, but not because we see some tan colored soil by the only substantial atmosphere retained by any natural satellite. Its composition appears to be primarily methane with perhaps some ammonia. The molecular weight of methane is high enough that unlike hydrogen or helium, a small world can retain large quantities of it. It is through that this atmosphere is very similar to the original atmosphere of Earth before blue/green algae changed the atmosphere to an nitrogen and oxygen rich air.
Companions
Companions are bodies which orbit a third body yet are linked together in various odd ways.
Saturn has two moons Epimetheus and Janus which both orbit Saturn on opposite sides of a thin ring. Because one of the moons is an orbit which is closer to Saturn, it travels faster and eventually overtakes the outer moon. As it gets close the outer moon decelerates the inner moon while the inner moon accelerates the outer moon. The two moons exchange orbits with the prior outer moon now on the inside and vice versa.
Earth has a companion as well, a small asteroid called Cruithne (pronounced croo-en-ya - a Celtic hero). Although its orbit is tipped steeply to the Earth's orbit, it has a semi-major axis that AVERAGES about 0.9999 AU. The Earth is so large that it scarcely is affected by Cruithne's minuscule gravity. What happens to Cruithne is quite something else. When Cruithne is slightly inside the Earth's orbit it catches up and the Earth slingshots to a slightly outer orbit which causes it to start to lose ground to the Earth. Eventually it loses enough ground that Cruithne is overtaken by the Earth at which time the slingshot works in reverse pulling Cruithne into an orbit closer to the Sun and speeding up the asteroid. If you counted the Earth and the Sun as a fixed line, Cruithne would trace a horseshoe pattern with respect to the Earth. It travels on the inside of the shoe when it is catching up and the outside of the shoe when it slows down. The whole cycle takes about 4 centuries.
Co-planets
When a satellite is extremely massive with respect to its planet, it really forms a binary system with both bodies revolving around a common center of gravity (the barycenter). Most satellites are very small with respect to the planet. Ganymede is the largest satellite in the solar system, but Jupiter mass is 12,817 times that of Ganymede. The pull of Ganymede is trivial compared to the pull of Jupiter on Ganymede. Two planets have satellites which are very large relative to the primaries Earth and Pluto. The Earth is only 81 times as massive as the Moon. Pluto is about 8 times as massive as Charon.
Pluto and Charon rotate about their barycenter which is 1/8 the distance from Pluto to Charon. The barycenter lies outside of Pluto.
The barycenter of the Earth and the Moon lies about 4740 kilometers from the center of the Earth in the direction of the Moon. If you didn't see the Moon from Mars, you could easily deduce it was there because the Earth moves about 1/3 of it diameter side to side every 29.5 days. This also greatly complicates calculating where the planets are because we cannot ignore our movement around the barycenter for precise calculations.
Asteroids
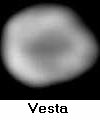
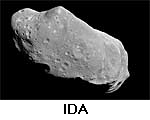
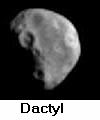
Vesta is one of the largest asteroids but as you can see from this photograph, it is not spherical like planets or larger satellites. Residing as it does inside the asteroid belt, even if it had original cooled as a sphere, collisions with countless smaller asteroids would have ensured that it was pockmarked and gouged.
Dactyl is a satellite of Ida. They were the first such pair discovered. Since their discovery other pairs have been discovered. At least a few of the asteroid pairs are contact pairs. They rotate very slowly because their gravitational pull on each other is very small.
In the year 2004 Toutatis will come within 6 Moon distances from Earth. Currently there is a lot of activity trying to establish the orbits of asteroids which cross the orbit of Earth. Unlike long term comets which we may not see until it is too late, it is entirely possible to identify any potentially dangerous Earth crossing asteroids early enough to do something meaningful to pull the asteroid out of a dangerous collision.
It is rather frightening to realize how small a civilization ending asteroid can be. The asteroid which killed the dinosaurs 65 million years ago was not some huge near planet sized body, but something on the order of the size of a mountain. The effects of such a collision are far ranging. There is no place on earth that would escape if a ten mile diameter asteroid hit us. It would compress a shaft of air ten miles across until all the air became nitric oxide - the basis of nitric acid. If the asteroid hit land a huge crater would be dug and ejecta thrown up in the air blotting out the Sun for several years. During this time nothing would grow. Hitting a ocean would have even more terrible effects.
The fact we haven't been hit in 65 million years is not too much comfort to those of us who watched Shoemaker Levy 9 crash into Jupiter.
Comets
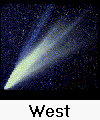
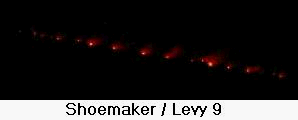
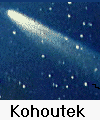
Comets are planetesimals composed largely of volatile materials. When a comet comes close enough to the Sun it often out gasses a long and brilliant tail. Comets travel in extremely elongated elliptical orbits. At the most distant point in the orbit, the comet moves so slowly that it may stay out there thousands or even millions of years. When they move into the solar system, the relentless gravity of the Sun accelerates day after day for decade upon decade. They can be traveling at speeds up to 70,000 miles per hours when they round the Sun.
Sometimes long term comets happen to pass near a gas giant - particularly Jupiter. Either the comet's speed will be increased or slowed by the encounter. If its speed is increased it will pass the Sun with excess velocity that will cause the comet to leave the solar system forever. If it slows down, the comet may become a inner comet like Haley's which has a period under one hundred years. There are two principle reservoirs of comets, the Kuiper Belt (home of the transneptunian objects) just outside the orbit of Neptune and the Oort Cloud about 50,000 AUs (about 8/10 of a light year) from the Sun. The Kuiper belt is a disc similar to the asteroid belt but filled with comets. The Oort cloud is spherical. The Oort belt is so far from the Sun that occasionally the Sun and another star pass close enough that the other star pulls comets of of the Oort belt. Some are lost to the solar system forever but other are started on their slow way into the inner system.
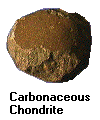
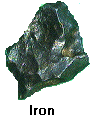
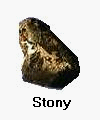
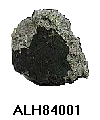
Debris from comets which have melted and released their stony components, as well as broken pieces of asteroids and occasionally, pieces of planets ejected when comets crash into the planets are scattered all over the solar system. Most of then are dust particles or sand like specks. However, larger pieces exist.
If a meteor hits the Earth's atmosphere it burns up from friction. If the particle is large enough to hit the ground it is called a meteorite. Roughly 100 tons of meteors fall on the Earth each and every day.
Meteorites come in three basic forms, ones which are largely iron come from the core of some proto-planet, ones which are are stony or a mixture of stone and iron which come from the collision of asteroids, and carbonaceous chondrites which are composed of carbon compounds. The meteorite ALH84001 is believed to have been chipped off Mars and eventually hit the Earth. ALH84001 caused quite a stir when scientist thought they detected fossil Martian bacteria on it. Such claims are very muted now. The bacteria fossils could have been from Earth or simply have been crystalline deposits.
Following the paths of melting comets, the density of particles can become unusually high. These create the so called meteor storms when the Earth orbit interests the orbit of such a comet. For example, Comet Tempel-Tuttle is the source of the swarm of meteors we call the Leonids. Swarms seem to come from a single point in the sky called the radiant. This radiant stays located' basically in a constellation and gives the swarm its name.
Basic Astronomy (Session Two)
Stellar Systems
The Big Bang
The universe formed some 12.5 billion years ago. Much is speculation, but somehow from a tiny speck everything including space, time, matter and energy unfolded into something that became recognizable as an early version of the universe we see about us today. Initially, the temperature was too intense to allow matter to condense from energy. All of the energy was in the form of fierce gamma radiation.
After expanding for many thousands of years, the temperature of the Universe had cooled to the point where gamma radiation could form neutrons, protons and electrons. Almost all of this matter was in the form of hydrogen (91+%) and helium (8%) and less than a percent lithium, an isotope of hydrogen (deuterium) and an isotope of helium (helium 3). Almost no other elements were created at this time. It is a matter of debate whether primordial black holes were also created. The forces were enough so that dense knots of matter could create black holes. These black holes may be the "seed" around which galaxies formed.
The Universe Today
Today large clouds of gas exist throughout the universe. Most of it is simple atoms, but some of these clouds contain simple molecules and dust. Most of it has collected in and around galaxies. While we see star formation throughout the universe, and we see the absorption of smaller galaxies when the encounter larger galaxies, we no longer see the formation of new galaxies.
The nature of the interstellar gas is very different today from the original gas. While hydrogen and helium still abound, other elements can be found in densities as high as 7%. This has profound consequences for the type of stellar systems that can form. Most importantly, heavy elements allow rocky planets such as the Earth to form. These new elements came from the transmutation of elements in the hearts of first generation stars. Elements up to iron in weight can be formed in normal stars and ejected into space as solar winds and exploding shells when the stars reach the red giant stage. Elements heavier than iron are created and distributed by a much more dramatic process - supernovas.
Galaxies
Edwin Hubble assigned a naming convention to galaxies which remains in use today. Galaxies come in three main forms, irregular galaxies with shapes that are amorphous, elliptical galaxies with a large core and almost no disk, and spiral galaxies which come in two forms, those with a large central cylinder of stars (barred) and those where the spirals go all the way to the core.
The shapes of galaxies appears to start as spirals of one sort or the other. Over time galaxies pas near or actually through each other. Currently, a small galaxy [the Sagittarius Galaxy] is colliding with our Milky Way. When they do this the smaller galaxy loses many of it stars to the larger galaxy. If the smaller galaxy comes into too close a contact it may be simply swallowed by the greater galaxy. If it is somewhat farther away it may escape badly tattered as an irregular galaxy. This is what seems to have happened to the Large and Small Magellanic Clouds. After swallowing enough smaller galaxies, the spiral shape disappears and the galaxy assumes an ever more elliptical shape.
The Great Nebula in Andromeda is a classic spiral nebula. At the leading edges of reaching rotating arm, a wave of gas compression occurs triggering areas of star formation. Andromeda is nearly edge on in this image but it would look like M74 if we saw it face on.
Virgo A also known as M87 is many hundreds the times the mass of our own galaxy. Trillions of stars are believed to populate this great object. Virgo A is the largest of the galaxies in the so called Realm of the Galaxies which spans parts of Virgo, Coma Berenices and Leo. This area has more galaxies to seen than it has stars visible to your eye. While it looks rather like a globular cluster, it is billions of times as large.
NGC1300 is a clear example of a barred spiral. Unlike a traditional spiral, the swirling arms star at the ends of a cylinder of stars which extends for many tens of thousands of light years from the center of the barred spiral.
Clusters
Open clusters of stars are formed in a common stellar nursery. In time birthing grounds like M42 and the Omega Nebula in the southern hemisphere will drive out gas which has not been included in the newly formed stars. In some open clusters like the Pleiades traces of the gas still can be seen in photographs sensitive to blue and ultraviolet light. [This gauzy gas is not visible to the human eye]. When the stars were formed they were densely packed. However they each had their own motions which over time causes them to disperse.
Globular clusters are effectively satellites of the galaxy in which they reside. They travels as units into the central areas of the galaxy on orbits somewhat like comets around the Sun. Stars in globular clusters are quite unlike stars in the main disk of the galaxy. Main disk stars like the Sun carry a great deal of heavier elements (metals to astronomers no matter what the chemists call them). Sun like stars are called Population I stars. Stars found in globular clusters lack more than a tiny percentage of heavy elements and form the Population II stars. The thin halo of stars outside the plane of the galaxy are also Population II stars. Population II stars formed before there had been many supernovae to create heavier elements. They are first generation stars for the most part. Population I stars form in the wake of supernovae and contain the heavier elements.
As the globular clusters orbit about the central core, they tend to be densest near the core and more sparse farther out. In our galaxy, the great concentration of globular clusters in Sagittarius confirms that this constellation harbors the center of the Milky Way. Further tests have shown that the exact center is a small volume in Sagittarius with at least a mass of 2 billion Suns. This dense area is believed to be a black hole and is called Sagittarius A*.
Nebulae
An emission nebula is gas excited by ultraviolet radiation from fierce new blue violet stars. This is the same conditions which occur in fluorescent and neon lamps. This type of nebula is typified by M42, the Great Nebula in Orion. The Trapezium as well as many unseen embedded stars provide the' sources of ultraviolet radiation.
An absorption nebula is a mixture of gas and particularly dust dense enough to absorb, redden and even blot out light. These cause dark nebulae like the Horse head and the Coal Sack. They cause dark areas like Sagittarius and the Sombrero. However they do not include "empty lanes" in the Milky Way.
Sometimes an nebula can have regions which emit while other regions absorb. This is the case in the Horse head Nebula shown here. The dark regions are areas where light has been absorbed so heavily that the area looks like a dark cloud. The bright regions are where hydrogen gas is fluorescing emit a reddish frequency called the hydrogen beta line. Near the edges of the dark regions areas absorb much but not all of the light and it is possible to use a spectrograph to determine the clouds chemical make up.
When light from a foreground stars shines on background clouds, a reflection nebula is formed. In some cases, the foreground star can be hidden by an absorbing nebula. Reflection nebula can sometimes be precisely mapped and measured by timing the pulses of light from a variable star or a supernova.
Planetary nebulae arise when an aging stars sheds shells of gas as the fusing of hydrogen leaves the core and moves towards the star's surface. Large explosions (but not as large as supernovae), progressively strip the star of material. If the star manages to shed enough material, the it will end up as a white dwarf with a ring about it which grows year by year. Eventually these rings become so large and thin that they are no longer illuminated by the hot central white dwarf.
Star Formation
Stars are continually being formed from the huge reservoirs of hydrogen gas the fill the galaxies. It was once thought that gravity played the role of "gas compressor", but we now know that there hasn't been time since the formation of the universe to have had many clouds compress naturally into stars. A triggering event is required. The two principle events are density waves and supernovae.
Density Waves
The center of every galaxy appears to contain a black hole. This is by no means certain, but something large and dense exists there. Lines of magnetic force stream outwards and are bent along the leading edges of the galactic arms. This creates a density wave which sweeps up and compresses hydrogen and helium along with any other elements which may be in the region. Although we cannot look down on the Milky Way to see such area, we can see similar areas in thousands of other galaxies. Along the leading edge of their arms, young fierce glowing blue white stars abound, a sure sign of star formation.
Role of Supernovae
"We are such things as dreams are made on" said Shakespeare. I wonder what he would have said if he realized that it is also quite literally true that once our very elements were forged in the hearts of the largest stars. Look at the Crab Nebula as the explosion which tore it apart sends material through space. However, the material which pours out of a supernova is not just the hydrogen and helium which formed the star but nitrogen, oxygen, carbon, silicon, sulfur, magnesium, neon, iron and in fact to some degree or other every element in the natural world.
One role of a supernova is to create the elements from which Population I (metal rich) stars are formed. These are the stars that can have rocky, watery worlds where life can form. The other crucial role that supernova play is as another source of gas compression and the triggering of new stars. Like density waves, the bow wave of a supernova explosion pushes everything before it and compresses gas until its own gravity can take over forming a new set of stars.
Hertzsprung Russel Diagram
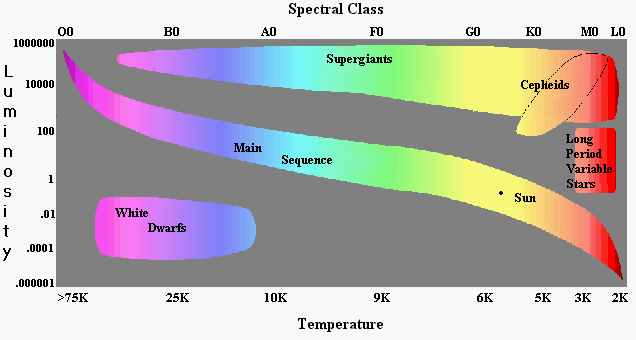
Stellar Luminosity
The luminosity (the total emitted energy) of a star is directly proportional to the fourth power of it mass. To maintain this power output, the star must consume its fuel proportional to its fourth power as well. If one main sequence star is 3 times as massive as another star, it will shine 81 times as brightly. It also fuses its fuel 81 times as rapidly. As stars leave the main sequence this relationship is disrupted.
The term luminosity is the preferred to describe the brightness of a star. For historical reason, the portion of a star's spectrum that lies in the visual range is measured by a magnitude scale. Stars of the first magnitude seem to be twice as bright as those of the second magnitude which in turn seem to be twice as bright as those of the third magnitude. In fact, a closer relation ship is that every five magnitudes in brightness represent a 100 fold change in luminosity. Luminosity is measured directly. Magnitude is measured on an inverse logarithmic scale. Larger magnitudes mean dimmer stars which is counter intuitive. Larger luminosities mean brighter stars exactly as you would think.
Do not confuse apparent luminosities (or magnitudes) with absolute luminosities (or magnitudes). Apparent brightness depends on how a star looks to us on Earth. Absolute brightness depends on how bright a star would be at the standard distance of ten parsecs (33.26 light years).
Stellar Lifetimes
The time that a star spends on the main sequence is INVERSELY proportional to the cube of its mass. This is a direct result of the luminosity relationship we just discussed. Since a stars luminosity (and hence its rate of fuel consumption) is proportional to the fourth power of the mass but its mass is only the first power, stars have a lifetime which is proportional to M/M4 or simply M-3.
Large stars have very short lifetimes. A maximal sized star of about 100 solar masses will live 1 MILLIONTH as long as the Sun. A minimal sized star of 0.08 solar masses will live 1950 times as long as the Sun. Since the Sun will live about 10 billion years, the largest stars burn out in just about 10 THOUSAND years but smallest stars will live 19.5 TRILLION years.
Stellar Classifications
New Stars
When stars coalesce from interstellar gas clouds, their temperature and pressure rise from frictional heating and gravity. Once nuclear processes begin gas already falling in from the spinning disk collides with gas expanding from nuclear fusion. One way that Herbig-Haro stars relieve this problem is to eject mass at the poles of the new star.
Young stars have yet to achieve hydrostatic balance between the rate of energy production and the size of the star. As much as ten times the material that will eventually form the finished star exists in the new stellar system. This material must be driven back into the interstellar medium. Stars in this stage of development are called T-Tauri stars.
Brown dwarfs weighing between 0.01 and 0.08 stellar masses are neither true stars nor planets but intermediate objects. They radiate in the infrared. Most of their heat comes from gravitational contraction. However, sometimes their central cores are hot enough to fuse deuterium, lithium or beryllium. These elements fuse at a temperature several million degrees cooler than the minimum required for hydrogen fusion. However, there are so few of these atoms, that they are unlike to encounter each other in a core that is largely hydrogen and helium. When these elements do fuse, they expand the core cooling it enough to shut down the reactions. far between to really ignite a star.
Once a body of hydrogen reaches 0.08 solar masses, it has enough material so that gravitational contraction will raise the central core to 15 million degrees. Hydrogen begins to fuse. A true star is born.
When the new star has a mass between 0.08 and 0.4 solar masses, it forms a small dim red dwarf star. Of the 100 nearest stars 92 are red dwarfs. They form in great numbers but their total luminosity is so low that galaxies seem blue white. Indeed, Proxima Centauri, the nearest star to the solar system is 13th magnitude - no brighter than dim little Pluto.
Most normal sized stars are the so called main sequence dwarfs. They are in the spectral classes K, G, F and A with masses between 0.4 and 3.3 solar masses. The term "dwarf" is unfortunate because it seem to imply a star of small dimensions. In fact they are much larger and brighter than an average star. For example the Sun is a yellow G2 dwarf, yet of the 100 nearest stars only 3 are a bit larger and another is just a bit smaller. 95 stars have diameter which are less than 60% of the Sun and masses which are less than 40% of the Sun. No nearby star is really large, although Sirius is almost twice the mass of the Sun.
Some orange, yellow, white (green) stars fall into a category of sub-giants. Sub-giants are large stars which are in the process of leaving the main sequence. These stars swell as the hydrogen fusion shell approaches the surface. Most of these stars are variables.
The largest main sequence stars are the blue giants. They are between 3.3 and 100 solar masses. While they are called blue giants, they can be blue, violet or even ultraviolet in color. These stars are extremely bright and short lived. Of the roughly 6000 stars that can be seen by the human eye, all but 50 are either red or blue giants. Blue giants of necessity are all very young stars. Some of these blue giants become unstable - like Dshubba and Gamma Cassiopeia - throwing off huge shells of gas and briefly becoming very bright. A few actually become supernovae without first becoming red giants.
Red giants posed a paradox to early astronomers. They were very red (hence they were cool) and they were very bright (which seemed impossible - because the black body laws [which we shall learn about in the Physics Section] say the red objects emit light dimly). Finally, astronomers realized that a star with a very low brightness per square meter could actually put out a huge luminosity if its surface area was enormous.
Red giants have HUGE volumes although they have low density. A typical red giant like Antares or Betelgeuse will have a volume as large as the orbit of Mars. The largest known red giant VV Cassiopeia is calculated to have a diameter as large as the orbit of Saturn.
Red giants are aging stars which have converted a large portion of their hydrogen to helium (typically 40-50%). As the core fills up with helium "ashes" the fusion zone approaches the surface. However at some point the gas above the star has too little remaining mass and the star stops being stable and begins to swell. The swollen star emits more light that before cooling it at a new less healthy stage. Red giants with lower mass (such as the Sun will become) will eventually simply become white dwarfs. High mass red giants are rapidly on their way to becoming supernova.
Stellar Instability
Variable stars
Eclipsing binaries are binary stars have the plane of their orbit edge on to the solar system. As the stars revolve around their barycenter they will regularly pass in front of one another. Since at least some of the total surface area is masked, the luminosity will drop. If one star is much brighter than its companion, there will be alternating large and small dips in the luminosity. By timing the dips precisely and determining the stars mass and velocity by applying Newton's laws of gravitation, it is possible to determine the diameters of the stars very accurately.
Flare stars appear to change more profoundly than they really do. All main sequence stars appear to emit flares. Against a bright star such as the Sun, Sirius or Rigel, a flare is lost in the overall brightness of the star. Against a dim red dwarf however, the flare can actually be brighter than the rest of the star's surface. All stars have flares where a pocket of overheated gas erupts at the surface. Momentarily, the star emits radiation of shorter wavelengths (blue, violet, ultra violet and x-rays). On a moderate star like the Sun, a flare tends to fade into surface brightness. Flares are unnoticeable on large blue stars. However, on a small red dwarf, a flare can actually be brighter than the star itself. For periods of a few minutes to a few hours the star may brighten several magnitude. Some amateurs watch a collection of red dwarfs looking for these flares.
Certain yellow orange sub-giants (called Cepheid variables) pulsate in a very regular manner. It is possible to determine exactly how far these stars are from the solar system by timing the pulse rate. What makes these Cepheid variables unusually useful is that they are bright enough to be seen in distant galaxies.
Hydrostatic balance is the balance between the expanding forces from the heat produced by fusion and a compressive forces from gravity. Imbalances between the expansion and compression can cause pulsations. These stars expand when they are hottest, emit radiation more rapidly when they are inflated, cool and contract in a cycle. Cepheid variables are examples of pulsating stars.
Stellar Deaths
Supernovae are the deaths of very large stars. Stars which start out at least 10 times the mass of the Sun cannot shed enough mass by ejecting shells by the time their core reaches 1.4 solar masses (Chandrasekhar's limit) [details to follow in Physics]. This results in an enormous explosions where all the elements of the periodic table beyond the first groups are produced. Supernovae can outshine their galaxy (billions and even trillions of star power) for a few weeks. Even this most titanic of nuclear explosions does not totally destroy the star. A core of compressed material remains. If the core is less than 1.4 solar masses it creates a white dwarf. If it is between 1.4 and 3 solar masses it forms a neutron star. More than 3 solar masses results in a black hole.
White dwarfs can result from supernovae, but they also are the end product of stars which go through the red giant stage without going supernova. The sun will someday become a white dwarf after it swells into a red giant stage. You can see a white dwarf at the center of the Cat's Eye nebula.
White dwarfs no longer fuse hydrogen into helium. The core is composed of helium or some heavier element (usually, carbon, oxygen, neon, silicon, magnesium or sulfur). Since there is no steady source of fusion energy, white dwarfs slowly cool down eventually become cold inert [hundreds of trillions of years] black dwarfs. No white dwarfs is believed to have entered black dwarf stage yet.
Astronomers used to think that nova and supernova were differing degrees of the same thing - stellar explosions. However, they are really quite dissimilar. Supernovae are titanic explosions which rip stars apart scattering elements into the universe. Novae are recurring small explosions which leave their "star" intact.
Novae are white dwarfs or neutron stars in close orbit around a main sequence star. The fierce gravity of the burnt out star strips the outer layers of hydrogen from the main sequence star. When enough accumulates on the burnt out star, a hydrogen bomb type explosion takes place.
We have already seen that neutron stars are supernovae remnants where the core is greater than 3 solar masses. These objects are very odd things indeed. In "normal" white dwarfs, the elements left after the supernova explosion are left as a plasma (sort of a gas where the electrons have been stripped away). The white dwarf does not have fusion energy to hold the star up from collapse, but the "electron pressure" (like charges repel) keeps the white dwarf steady at about the size of the planet Earth in diameter.
All this changes in neutron stars. Once the mass reaches 1.4 Sol, the gravity becomes so intense that the electrons are dragged kicking and screaming into the core. They get squished into the protons (positively charged nuclear particles) neutralizing them and becoming neutrons (uncharged nuclear particles). The star loses the pressure of the "degenerate electrons" and it collapses into a ball about 10 miles in diameter spinning at hundreds and thousands of times per second. The surface of a neutron star spins very near the speed of light.
Effectively, this neutron star is a single giant (fiercely radioactive) atom. It is very nearly the densest object in the universe. A sugar cube chunk of this stuff would weigh more that Mount Everest.
Pulsars (a type of neutron star) spin extremely rapidly. Near their poles, they emit charged particles at very near the speed of light. Think of them as swizzle sticks spinning around blindingly fast. The swizzle sticks of charged particles sweep up and stir around the gas in the system they reside in causing a form of electromagnetic radiation. Some of this is in the radio frequencies and the rest in higher frequencies up to visible flashes. If the beam of charged particles is lined up in the direction of the Solar system, the electromagnetic radiation will flash on us. When these very regular flashes were first detected many astronomers suspected they were artificially produced by alien species.
Supernova remnants greater than 3 solar cannot remain stable at the neutron star stage. They become the most exotic of all stellar objects - black holes. Gravity again begins its relentless pull. The gravity reaches a point where no particles, not even light can escape because they would have to travel above the speed of light (the universal maximum) to leave the ex-star. [There is an odd form of radiation (Hawking radiation) which can leave the event horizon of a black hole through quantum mechanical processes but we will not discuss it here].
For our purposes, the event horizon marks the point where anything that enters the black hole cannot leave. There is a false belief that black holes are all powerful vacuums which slurp anything and everything into their maw. This is not so. For example if you squeezed the Earth into a black hole (an event horizon about the size of a marble), and stood at a distance of 6,400 kilometers from it (our current distance from the center of the Earth) the gravity would be exactly 1 G. The field would only become great as we came very close to the black hole.
Quasars are extremely bright objects which can be seen across the universe. The only known source of such power would be a huge black hole swallowing the gas from stars unfortunate enough to get too near the black hole. The light is not emitted by the black hole itself, but a disk of material spiraling into the black hole. Quasars normally have long jets of material shooting out at nearly the speed of light. These jet can be luminous for light years.
Active galactic nuclei are suspected of containing black holes in their centers. In fact some theories say that all galaxies arose around a central black hole formed at the big bang [a pure guess so far]. Those which are so suspected have something very energetic at the core.
Seyfert galaxies have centers which seem very much like quasars without their jets. They appear to be ex-quasars or quasars which no longer have enough nearby gas to power the quasar. The heart of our galaxy is a black hole located at the object we call Sagittarius A*. It looks as if the Milky Way is (or at least was) a Seyfert type galaxy. The central black hole solar masses some 2-3 million solar masses. We can see stars orbiting Sagittarius A* in as little as decades.
Definitions
- Asteroids
- Asteroids (sometimes called planetoids) are planetesimals which orbit a star. Ideally, all asteroids would be planetesimals, however some larger asteroids are actually worlds. The dividing line is an arbitrary 1000 km.
- Dwarf Stars
- Dwarfs are regular stars like the Sun which have modest masses and modest volumes. Stars which are not some sort of "giant" are called dwarfs no matter what their size. super dense star is called a white or a black dwarfs.
- Giant Stars
- Giant Stars have volumes many thousands of times that of the Sun. Some "sub-giants" and "blue giants" have masses much greater than the Sun, but volumes which are not radically larger than the Sun.
- Main Sequence Stars
- Main Sequence Stars are huge bodies which derives the vast majority of their energy primarily from fusing hydrogen to helium. Main sequence stars are in hydrostatic balance between the forces of gravity and nuclear fusion. Stars too young to have achieved this balance throw off huge amounts of material via jets and fierce solar winds. Stars that have used up their hydrogen fuel supply swell enormously.
- Planets
- Planets are full sized spherical worlds which orbit a star. See rogues and asteroids.
- Planetesimals
- Planetesimals are bodies which is too small to attain spherical shape simply through their own gravity. A planetesimals melted by passing too close to a star and becoming spherical due to surface tension (a result of electromagnetic force) does not count, because the forming was not done primarily by gravity.
- Rogues
- Rogues are suspected (but unproven) worlds like planets that do not orbit stars. These are believed to be ejected from star systems as the systems grow older. See Planets.
- Satellites
- Satellites (often called moons) are either worlds or planetesimals which orbits a planet.
- Worlds
- Worlds are bodies large enough to be pulled into roughly spherical shape by their own gravity. All stars fall within this definition as do major planets and large moons.